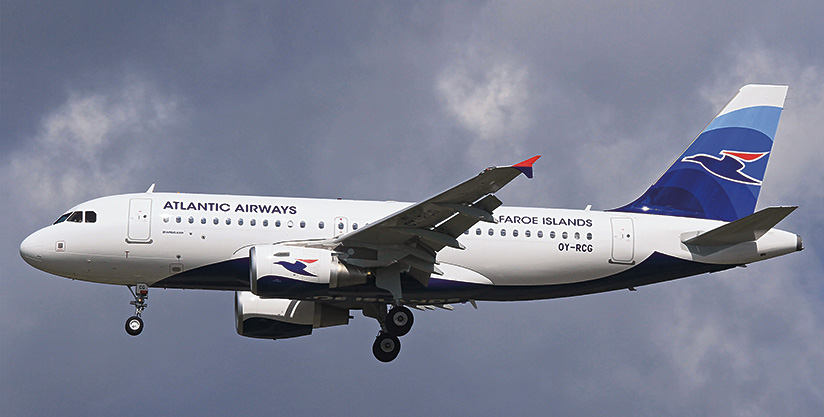
In March 2012, Atlantic Airways, the flag carrier of the Faroe Islands, became the first European airline to use required navigation performance with authorization required (RNP AR) 0.1 procedures on a commercial flight.1
“At the time of landing — around 4 p.m. — the western Atlantic winds had spread a mass of low-level clouds above the channels and fjords of the Faroe Islands. We could only see the spines of the mountains,” said Andrea Artoni, an aeronautical journalist, aviation consultant and a former air traffic controller who was aboard the delivery flight of the Atlantic Airways Airbus A319. The new aircraft was equipped with the RNP navigation system.
“The pilots consulted the approach chart. Tension was palpable. The weather conditions forced the pilots to use, starting from the very first flight, the special instrument approach procedure which determined the decision of the airline to purchase exactly that aircraft with exactly that equipage,” said Artoni.
The equipment aided a perfect landing and since has enabled the airline to improve airport access and service reliability at its operationally demanding Faroe Islands base. The RNP navigation system installed on the Atlantic Airways A319 uses sophisticated positioning equipment to enable pilots to conduct approaches and takeoffs in challenging weather conditions that are typical in the Faroe Islands, an archipelago under Danish rule situated between the Norwegian Sea and the North Atlantic Ocean. The system also guides pilots along the non-linear approach path to Vágar Airport, necessitated by the high terrain at either end of the runway.2
Efficient Navigation
RNP is a category of procedures under performance-based navigation (PBN), an overarching concept comprising different levels of precision in navigation procedures without specifying the technology used to achieve them. Also included under PBN is area navigation (RNAV), the broadest category of PBN procedures. The main difference between RNAV and RNP is that RNP equipment includes alerting and monitoring systems allowing pilots to constantly monitor, on the flight management system display, the RNP precision value in nautical miles. Furthermore, RNP instrument approach procedures are flown with the autopilot selected and using only satellite signals. PBN allows aircraft to fly flight paths more precisely than previous standards, and without the necessity of having a direct link between a ground-based navigation aid (NAVAID) and an aircraft navigation system, thus allowing improved operational efficiency and better utilization of available airspace.
PBN can be considered a framework for “defining a navigation performance specification along a route, during a procedure, or in airspace within which an aircraft must comply with specified operational performance requirements. It provides a simple basis for the design and implementation of automated flight paths and for airspace design, aircraft separation and obstacle clearance. It also offers a straightforward means to communicate the performance and operational capabilities necessary for the utilization of such paths and airspace. Once the performance level (i.e., the accuracy value) is established on the basis of operational needs, the aircraft’s own capability determines whether the aircraft can safely achieve the specified performance and thus qualify for the operation.”3
Under PBN, generic requirements are defined on the basis of operational requirements. “Operators then evaluate options in respect [to] available technology and navigation services, which could allow the requirements to be met. An operator thereby has the opportunity to select a more cost-effective option, rather than a solution being imposed as part of the operational requirements. Technology can evolve over time without requiring the operation itself to be revisited as long as the requisite performance is provided by the RNAV system.”4
The expected benefits of widespread PBN usage include reduced fuel consumption and greenhouse gas emissions and relief of congested airspace. A relatively large proportion of the global airline fleet already is equipped with PBN navigation systems. According to Marcello Astorri, an air navigation systems consultant and former airline pilot, “The world’s 10 largest airlines have 97 percent of their fleets equipped with RNAV capability, while 47 percent of their fleets are equipped with RNP systems.” The problem, however, is that the operational approvals to actually navigate in accordance with PBN are still relatively few. “Only 23 percent of the world’s 10 largest airlines’ fleets have operational approvals to fly RNP approach procedures in accordance with ICAO’s [International Civil Aviation Organization’s] PBN Manual,” Astorri said during a technical seminar in Rimini, Italy, in September. The seminar was organized by Artoni as part of AIRET, a technology trade fair.
Safety Implications
The enthusiasm of airlines to use PBN procedures, the legislative activity of regulators with regard to PBN and the consequent need of air navigation service providers (ANSPs) and airports to adapt to a new operational and regulatory environment should be managed with a focus on the safety implications of such a technological, operational and cultural shift in air navigation. “While it is true that technological innovation, both at hardware and software levels, on the ground and airborne, offers a real help for the increase of safety within all aspects of air navigation, it is also true that the introduction of new systems and procedures can create a situation of confusion and work overload to users, which can endanger the level of safety. Especially in cases of operational disruption, when the failure of automatic systems can create a situation of uncertainty, and operators can find it difficult to carry on their tasks, it can be complicated to understand what is happening and what to do once the disruption is over,” said Mauro Barduani, an air traffic control (ATC) officer and an air traffic management (ATM) and safety scholar.
There is a range of possible threats in the transition to PBN, and they are of concern to all stakeholders involved, namely air operators, ANSPs, airports and regulators.
The primary consequence of PBN implementation is a change in the airspace concept under which such navigation procedures are performed. “Validation of an airspace concept involves completing a safety assessment. From this assessment, additional safety requirements may be identified which need to be incorporated into the airspace concept prior to implementation,” said ICAO. “Four validation means are traditionally used to validate an airspace concept: airspace modeling, fast-time simulations (FTS), real-time simulations (RTSs), and live ATC trials.” If the changes in the airspace concept are consistent, then a combination of the four means may be necessary.
Airspace modeling is a beneficial first step because it provides some understanding of how the proposed implementation will work while not requiring the participation of controllers or pilots. “Airspace models are computer-based, so it is possible to make changes quickly and effectively to ATS [air traffic service] routes, holding patterns, airspace structures or sectorization to identify the most beneficial scenarios (i.e., those that are worth carrying forward to more sophisticated types of validation). Using a computer-based airspace model can make it easier to identify non-viable operating scenarios so that unnecessary expense and effort [are] not wasted on more advanced validation phases. The main role of the airspace model is to eliminate non-viable airspace scenarios and to support the qualitative assessment of further concept development,” according to ICAO.
An FTS is a more sophisticated assessment than airspace modeling, and it “returns more precise and realistic results, while still not requiring the active participation of controllers or pilots; however, in terms of data collection and input, preparation can be demanding and time-consuming,” ICAO said.
RTSs realistically replicate ATM operations and require the active participation of proficient controllers and simulated or “pseudo” pilots. “In some cases, sophisticated RTS can be linked to multi-cockpit simulators so that realistic flight performance is used during the simulation. One of the difficulties that can be encountered with real-time simulation is that the navigation performance of the aircraft is too perfect. Aircraft in RTS may operate with a navigation precision that is unrealistic, given realities of weather, individual aircraft performance, etc. In such cases, error rates from live operations are analyzed, and these can be scripted into the RTS,” reported ICAO.
Live ATC trials are used to verify operating practices or procedures when subtleties of the operation are such that FTS and RTS do not satisfy validation requirements.
The initial safety assessment for the airspace concept validation should be performed in parallel with the identification by all stakeholders of more specific issues associated with transition to PBN.
SOP Redesign
PBN procedures “improve the predictability and efficiency of the flight paths but require additional coordination and planning. … The increased predictability and consistency of the PBN transitions can in some cases limit the flexibility the controller has in providing vectors close in to the airport,” according to a GE Aviation white paper published in July.5
“From an airline perspective, flight crews must be provided with training and policies to ensure that they are aware of conditions where the PBN path places the aircraft within range to unintentionally capture the final segment,” stated GE Aviation.
The redesign of standard operating procedures (SOPs) will ensure compliance with the new PBN regulatory requirements — whichever these will be — as well as navigation within the required safety levels. “However, there may still be terrain-challenged airports where placing the PBN path within range of final segment guidance may be necessary,” the white paper said.
Regulatory Coordination
Regulatory adaptation and the definition of international standards is a long and delicate process, but it is necessary to ensure minimum levels of safety all around the world. The problem is not specifically that there could be differences in PBN regulation between different regions of the world. The issue is rather that in politically integrated regions like the European Union, where there are 27 national aviation authorities (NAAs), regulatory inconsistencies may be generated because it is a competence of the NAAs to implement legislation promulgated at Community level, including the PBN-related implementing rules under development. “So far, even if there exist some standards of reference, namely ICAO’s PBN Manual, several operators and countries continue to utilize their own ‘parameters,’ in particular with regard to maintenance regulations and minimum levels of service,” said Barduani.
It becomes necessary, therefore, that each ANSP not only complies with the regulatory requirements of the competent authority to which it is subjected, but also that it keeps up to date with the PBN-related regulatory differences existing among different countries, in particular those among the countries where the PBN operations are most frequent.
Workforce Resistance
“A reluctant behaviour on the side of the workforce [during] the evolution towards PBN concerns the natural human tendency not to be always available and open to technological changes, especially if such changes lead to increased workloads and reduced personnel for certain types of assignments, which could become completely automated,” said Barduani.
Clarifying to the workforce the reasons for change — operational efficiency and market competitiveness — can be useful in addressing the workforce’s reluctance to change. Such a response can be supported and complemented by a carefully weighed system of incentives targeted at individual workers demonstrating proactive adaptation to new technologies and practices. The basis of such a system of incentives may be the quantity and quality of observations made by the organization’s leaders with regard to how their PBN procedures are implemented.
Knowledge Gap
It is likely that before and at the beginning of the transition to PBN, an organization may not have the required technical knowledge to manage the transition. As a matter of fact, there is a need to retool flight crews, dispatchers, operational personnel and safety experts in air transport regulation and economics in light of PBN.
“The increased emphasis on training activities in the domain of PBN represents a significant evolution from the tradition of didactic training in air navigation,” said Astorri. “If we consider the intricacies of the new RNP approach procedures, which require higher attention from the pilot with regard to the fly-ability of the procedures and the increased need of qualifications released by the NAAs, the response of the operators will have to be focused on combining together a cross-cultural approach in PBN fundamentals, a new syllabus for RNP-rated flight crews to be conducted in a high-performance simulation environment and the organizational certifications.”
In addition to PBN-specific training, a further element that should be recognized is the need for more targeted training in threat and error management, crew resource management, and command and decision making for flight crews. “There is indeed a risk of increased complacency as pilots no longer actively use and search the inputs from the traditional navigation infrastructure and only need to concentrate on maintaining the precision of the on-board navigation system, which is ensured by the autopilot and the accuracy of satellite signals,” said Astorri.
Beyond the flying community, PBN-specific training also will have to be provided to ANSP personnel, aeronautical information services personnel, airport operators and military personnel, when necessary.
Traffic Increase: Airspace
The forecast increase of traffic in ATM systems represents a significant threat even if, as expected, PBN makes airspace management run more smoothly. During the transition to PBN, safety can be enhanced by the sharing of relevant information among key stakeholders. “Under SESAR [Single European Sky ATM Research], there are provisions for a SWIM (system-wide information management) ATM information model. SWIM has been designed to allow communication, develop programs, and transfer and share information in a simple way, in order to permit users to acquire data without the necessity of specific and deep knowledge of the system’s features. The concept is to create a ‘system of systems,’ and to reach this goal, it is necessary that all operators have access to a shared information and data system. From the perspective of ATM, widespread cooperation is essential to define optimal aircraft flight paths, thus allowing [ATM] to [transition] towards trajectory-based operations (TBO),” said Barduani.
Traffic Increase: Airports
Airports also will face traffic increases because PBN allows more reliable operations to and from a given airport and thus more movements to and from the same airport. “According to a forecast [by] Eurocontrol, approximately 20 percent of the future air traffic demand could be not accommodated, mainly because of the lack of capacity at European airports. Even if there is still capacity at other levels, like en route airspaces, there will likely be constraints at airport level, representing a real bottleneck for the whole system,” said Barduani.
In addition to the necessary infrastructural investments to increase capacity at airports, an airport collaborative decision making (CDM) platform can help the ATM network to plan in advance a strategic flow management and give precise timing to airports for the utilization of their infrastructure and services. “The concept is difficult to implement but allows for higher predictability and punctuality, permitting airports to be more flexible, use their infrastructure efficiently and on time according to the demand, reduce delays and taxi times, switch slots among flights, [and] plan in advance recovery actions in case of disruption,” said Barduani.
Safety Planning
Initial identification and assessment of risks, followed by their mitigation, should be the basis of further safety planning by all PBN stakeholders. Activities and time schedules to be used and respected should be identified; responsibilities among the members of safety teams should also be split. Objectives and targets of the safety action should be defined and reference safety indicators listed and outlined.
In the field of ATM, the safety indicators can be considered as the workload, the situational awareness, the losses of separation, the usability (the measure of the ergonomics and fitness for use of any work tool), the errors, the teamwork, the level of trust, the acceptability (level of trust in the effectiveness of a system or procedure for the execution of tasks) and the degree of skill degradation due to automation.
“Safety planning at the level of the single operator should also consider issues associated [with] the systemic interdependencies which characterize transition to PBN” said Michael Grüninger, an aviation safety consultant and formerly a flight inspector at the Swiss Federal Office of Civil Aviation. Among the systemic interdependencies which could give rise to safety issues, he listed a lack of cooperation among key stakeholders, a poorly defined and managed interface, a deficit of competences, a deficit in the visualization of the new system and an unsuccessful identification of the peculiarities, such as aircraft and approach design.
“An increased systemic complexity results in stronger interdependencies and an increasing amount of issues which cannot be solved on a stand-alone basis. What changes with PBN with regard to safety planning is an increased awareness of the implications of one’s decisions and actions in the context of an even more complex and interactive system. With the transition to PBN, there will be an even stronger need to make well-thought decisions coordinated among all players and stakeholders,” said Grüninger.
Notes
- As explained in “Preparing for PBN,” by Frances Fiorino in Avionics Magazine, February 2011, RNP AR “requires the highest level of navigation performance. … A value of ‘RNP 0.3’ means the aircraft is capable of remaining within 0.3 nautical miles to the right or left of center line 95 percent of the time within a defined containment area; RNP 0.1 means an aircraft must remain within 0.1 nm.”
- Shepherd, Lesley. “Atlantic Airways Airbus A319 First in Europe to Use RNP.” <www.eraa.org/publications/industry-news/872-atlantic-airways-airbus-a319-first-in-europe-to-use-rnp>.
- FAA. Roadmap for Performance-based Navigation – FAA – Version 2.0, July 2006.
- ICAO. Performance-based Navigation (PBN) Manual (Doc 9613 AN/937), Third Edition, 2008.
- GE Aviation. PBN to xLS: Implementation Today. GE white paper prepared by Naverus, Inc., 9 July 2012.