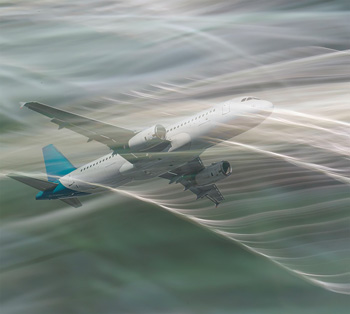
It seemed that airplanes arriving and departing from Will Rogers World Airport in Oklahoma City, Oklahoma, United States, on the morning of Aug. 3, 2012, would have few problems with wind conditions. The air was dry, and the surface weather map was devoid of any significant systems, typical of summer in the Southern Great Plains. Winds were light at the surface, running about 5 kt. And winds well aloft also were weak, less than 25 kt to 40,000 ft. So imagine the surprise when pilots ran into winds in excess of 40 kt just 1,600 ft above the ground. This was an example of what meteorologists call a “low-level jet stream” or a “low-level jet.”
Jet streams are fast-moving currents of air that have been likened to rivers in the sky. For many years, meteorologists only theorized about their existence. Driven by the inherent temperature contrasts on Earth and the effects of the planet’s rotation — the Coriolis effect — the air never is still. In the meteorologists’ view, fluid mechanics theory indicated that the air’s movement should be concentrated in some regions — these areas eventually were called jet streams.
Later, aviation provided the absolute proof. When airplanes started flying higher, particularly by World War II, pilots encountered the strong winds of the jet stream. These are the now-familiar high altitude jet streams that normally occur from 30,000 to 35,000 ft.
As the science of meteorology progressed, and the atmosphere was probed more thoroughly on a regular basis, other jet streams were discovered. Some were many miles up, on the threshold of space, but others were close to the surface. These are the low-level jets, and they are a particular concern for aviation given the potential for loss of control of an aircraft so near the ground.
A low-level jet stream is a wind speed maximum found within the lower part of the atmosphere. Its height can vary from 5,000 ft down to a few hundred feet above the surface. Wind speeds typically range from 20 to 50 kt, but have exceeded 80 kt in extreme cases. To be officially classified as a jet, the wind speeds above this low-level maximum must be relatively slower. However, operational meteorologists and aviation interests would be more concerned with any rapid increase in wind speed with height near the surface, regardless of the wind profile above it. Low-level jets can be several hundred miles long and tens of miles across.
For example, for a low-level wind of 50 kt at 5,000 ft to be officially called a low-level jet, winds would have to decrease to, say, 30 kt at 10,000 ft. But if the winds at 10,000 ft were 70 kt, the 50-kt wind at the lower height would not be a low-level jet. You would still have 50-kt winds at 5,000 ft, however, and that could cause problems for aviators.
Low-level jets are a result of dynamic processes in the atmosphere. Without going into the mathematics, basic physics shows that when strong temperature contrasts exist, winds increase. Fronts, which separate warmer from cooler air masses, are, by definition, regions where strong temperature contrasts exist. So low-level jets tend to be found near various fronts. Topographic barriers such as mountain ranges also affect airflow and can intensify low-level winds into jets.
Low-level jets pose a number of problems for aviation. These potentially dangerous conditions just above the surface can occur during the critical times of takeoff or approach to landing. As with upper-level jet streams, low-level jets produce turbulence and there also are rapid changes in wind speed (i.e., low-level wind shear) that can affect the handling of an aircraft. Head winds or tail winds, which affect the lift generated by the wings, can change suddenly. Strong, rapidly changing crosswinds can pose a major hazard for planes attempting to land. Even if the jet stream itself is located some distance above the surface, strong wind gusts can occur near the ground.
Whether strong winds from a low-level jet reach the surface depends on the structure of the lower atmosphere. Meteorologists call the layer of air in contact with Earth’s surface the boundary layer. If this layer is well mixed, then strong winds well above the surface can mix downward, at least in strong gusts.
Sometimes the boundary layer is separated from the atmosphere above it by a stable layer, usually an atmospheric inversion. This happens when there is dense, colder air near the surface and warmer air aloft. If a low-level jet is above the stable layer or inversion, strong winds will not make it down to the ground, but pilots will note a sharp increase in wind speed when they approach the jet stream.
Low-level jets can be detected by radiosondes, balloon-borne instrument packs that are launched twice a day from more than 80 sites in the United States and 900 stations around the world. As the instrument packs rise, they move with the air currents. By tracking the radiosondes, meteorologists can determine the direction and speed of the wind at various levels of the atmosphere. On some occasions, Doppler radar (ASW, 9/12, p. 24) also can detect low-level wind maxima.
Low-level jet streams are fairly common and have been observed on every continent. There are many different types of low-level jets and a variety of situations that produce them. To start with, we’ll examine jets that occur with well-defined synoptic situations — the weather features easily seen on standard weather maps.
The 0000 UTC Jan. 23, 2012, surface chart for the United States (Figure 1) shows a developing low pressure area in the middle of the country. The counterclockwise flow is producing strong southerly winds ahead of the cold front and a northwesterly flow of colder air behind it. A look at the wind field at 850 millibars (mb) (Figure 2), approximately 5,000 ft above sea level (ASL), shows two distinct low-level jets. Ahead of the cold front, we have a prefrontal, southerly jet.
The Little Rock, Arkansas, radiosonde sounding showed winds in excess of 40 kt at 1,600 ft, increasing to 60 kt at 6,500 ft. Strong wind gusts exceeding 40 kt were reported at Clinton National Airport in Little Rock, brought down to the surface in thunderstorm downdrafts. Looking further north, although the jet wind speeds were still strong, surface winds only gusted to 20 kt at Lincoln Capital Airport in Springfield, Illinois. Colder air near the surface blocked the stronger winds from making it to the ground.
Strong southerly jets are very common in the Great Plains from the United States northward into Canada. One contributing factor is the topography. The Rocky Mountains border the plains to the west. They provide a physical barrier to the airflow, thus concentrating the southerly winds into jets. Although these jets develop regardless of the moisture content of the air, winds from the south can transport moisture from the Gulf of Mexico well north. In addition to the direct aviation problems associated with these jets, strong-to-severe convection also can accompany them, especially in the spring.
A post-frontal, northerly low-level jet also can be seen in the Western Plains. Winds exceeding 50 kt were recorded for Dodge City, Kansas, in a sounding at a height of 1,600 ft. Although the air was cold, it was well mixed, and wind gusts of 33 kt were reported at the Dodge City Regional Airport.
An even stronger northerly low-level jet was recorded during the major cyclone in the western Great Lakes on Oct. 27, 2010 (ASW, 2/12, p. 47). Bismarck, North Dakota, was well behind the surface cold front and into the cold air. A low-level jet of 70 kt from the northwest was noted at 2,300 ft above the ground. Surface winds gusted to nearly 50 kt. Above the jet, winds calmed. The wind speed at 30,000 ft, standard jet stream height, was only 39 kt.
Looking again at the surface weather map (Figure 1) of the United States, meteorologists saw a ridge of high pressure wedging down the east side of the Appalachian Mountains. The clockwise flow around the center of high pressure was producing northeast winds along the East Coast. The Moorehead City, North Carolina, sounding showed a wind maximum of 24 kt about 820 ft above the surface. Winds above this were much lighter. This type of low-level jet in a cold, northeast flow is even stronger when there is a major low pressure area to the south.
At 0000 UTC Dec. 27, 2010, a major winter storm was affecting the New York metropolitan area (ASW, 2/12, p 47). The sounding taken at Upton, New York, showed northeast winds of 51 kt just 1,000 ft above the surface. Wind speeds peaked 4,000 ft up, at 77 knots. Winds lessened considerably above this. Wind gusts of 50 kt at the ground were measured at John F. Kennedy International Airport.
In addition to intensifying any preexisting jets, mountain ranges can produce their own low-level jet streams. Barrier jets are formed when at least part of the overall wind flow is perpendicular to a mountain range. In particular, cold air masses — which are very dense — are blocked by higher terrain.
With warmer air above, this lid of stability blocks the air from flowing over the mountaintops. Instead, the airflow becomes parallel to the mountain chain and accelerates into a jet. The highest winds are found on the windward side of the mountain range below the ridge peaks. Wind speeds can exceed 50 kt. Barrier jets have been noted in many locations around the world where there is a combination of cold air and mountainous terrain.
Another type of low-level jet stream is the coastal jet. Often measured along coastlines, they show strong temperature contrasts. In particular, along the western coasts of continents we usually find cold water due to prevailing cold currents and the process of upwelling, that is, cold bottom waters rising to the ocean surface. In the summer, the cool air over the water contrasts greatly with the warmer air over the land. There is also very often a capping inversion found at such locations.
The end result is often a low-level jet just off shore that flows toward the equator. Wind speeds of 20 kt to 40 kt are common, and they can exceed 50 kt. The jet core is often near 1,300 to 1,600 ft. Coastal jets are common along the West Coast of North America from California to Alaska. They also have been observed off the coasts of Chile and Peru, Spain, and southwest Africa.
The low-level jets described above are linked to easily identified weather systems or to various geographic or topographic features. Other low-level jets occur in much more innocuous situations.
The 1200 UTC sounding on Sept. 12, 2012, for Peachtree City, Georgia, (Figure 3) depicts a particularly deceptive phenomenon. In looking at the vertical wind profile, calm winds at the surface change to 21 kt by 1,200 ft above the ground. Then the winds decrease above that height, and remain remarkably light. At the surface, the region is under a high pressure area.
What is causing this low-level wind maximum? A look at the vertical temperature profile shows that the strongest winds correspond to the highest temperature reported. The temperature has, in fact, increased from the surface to this point in a classic example of the nocturnal inversion. This means that when the sun goes down, Earth’s surface radiates heat into space and cools quickly, more quickly than the air.
The air nearest the surface is cooled from below, from being in contact with the cooler ground. Thus, a surface-based temperature inversion has developed. Usually, the coolest temperatures occur near sunrise, about the time of this sounding. The inversion layer decouples the surface layer of air from the air above it, also removing the frictional drag found at the surface.
Without friction to slow the moving air, wind speeds increase and are maximized right at the top of the inversion layer. This wind aberration is called the nocturnal jet. Technically, this is not a “true” jet — without other atmospheric processes being involved, it will dissipate in the morning as the air warms and vertical mixing sets in. However, the decoupling effect itself is important, and the true low-level jets described above tend to be stronger at night and in the early morning.
Finally, aviators may ask, “Can low-level jets be predicted?” Southerly jets — the largest and strongest of the low-level jets — can be forecast by some standard computer models. A number of fine-scale models also have had some success in forecasting the occurrence of less-pronounced low-level jets out to 18 or 24 hours. Today, there are still large errors in height and intensity forecasts, however. Often these jets have to develop first and then be detected before simple continuity forecasts can be made. The Oklahoma City situation above would fall into this category.
Edward Brotak, Ph.D., retired in 2007 after 25 years as a professor and program director in the Department of Atmospheric Sciences at the University of North Carolina, Asheville.