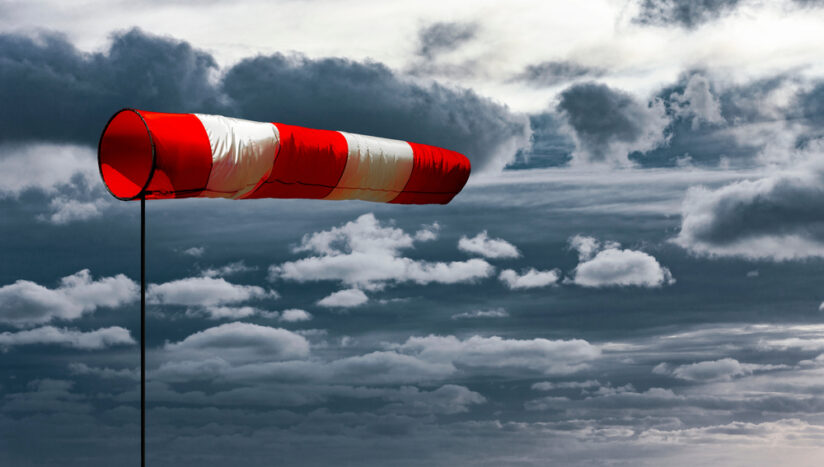
This article is the fourth in a series on landmark events in aviation since Flight Safety Foundation began in 1945.
An airliner approaches a runway as rain lashes the windscreen. Turbulence rocks the wings, and precipitation static hisses on the radio. Lightning cracks in the distance. The pilots see the airspeed increase — then drop off. The vertical speed indicator shows a steep descent. An aural alert sounds: “WIND SHEAR, WIND SHEAR.”
The captain, flying the aircraft, calls, “Wind shear, max thrust.” He thumbs the go-around button, disengages the autopilot. Adds power and pitches up. Then he follows flight director guidance as the aircraft climbs away. The first officer advises the tower that the aircraft is going around due to a wind shear encounter. Another aircraft a few miles behind requests a holding pattern to wait out the dangerous winds before attempting an approach.
What might have spelled disaster turns out to be a mere inconvenience. That’s because the crew knew what they might face, how to recognize it, and what to do about it. Automated systems backed up their training, warned them of the hazard, and guided them out of danger. They benefited from more than a half century of research on wind shear.
The U.S. Federal Aviation Administration (FAA) defines wind shear as a change in wind speed and/or direction over a short distance. Wind shear can occur at any altitude, but low-level wind shear causes the most concern for pilots. The FAA says four common sources for low-level wind shear include frontal activity, thunderstorms, temperature inversions, and surface obstructions.
Pilots watch for indications of wind shear that include:
- Airspeed variations of 15 kt or more;
- Vertical speed variations of 500 fpm or more;
- Pitch attitude excursions of five degrees or more;
- Glideslope variations of one dot or more; and,
- Stagnation or decrease of indicated airspeed on the takeoff roll.
According to the U.S. National Center for Atmospheric Research (NCAR), wind shear accidents have claimed more than 1,400 lives worldwide since 1943.
In 1964, as awareness of the problem grew, the International Civil Aviation Organization called for research on low-level wind shear.
The phenomenon was little understood prior to the 1970s. Accidents that increased concern included the June 24, 1975, crash of Eastern Air Lines Flight 66 at John F. Kennedy International Airport in New York. The Boeing 727, arriving from New Orleans, encountered a strong thunderstorm with heavy rain on final approach. The accident killed 113 people. A report by the U.S. National Transportation Safety Board listed the probable cause: “The aircraft’s encounter with adverse winds associated with a very strong thunderstorm located astride the instrument landing system localizer course, which resulted in a high descent rate into the non-frangible approach light towers.”
According to the FAA, the Eastern Flight 66 crash led directly to the development in 1977 of the low-level wind shear alert system (LLWAS). LLWAS gathers wind speed and direction data from pole-mounted sensors installed around the airport. When algorithms calculate the presence of wind shear, the system alerts air traffic controllers, who pass the information on to pilots.
Further research on the Flight 66 crash and other accidents identified a particularly dangerous form of low-level wind shear: microbursts. The U.S. National Weather Service (NWS) defines a microburst as “a localized column of sinking air, or downdraft, within a thunderstorm and … usually less than or equal to 2.5 miles [4.0 km] in diameter.” The NWS says wind speeds in microbursts can reach up to 100 mph and can cause major damage to homes and other structures.
University of Chicago meteorologist Theodore “Ted” Fujita led research into microbursts and other dangerous weather phenomena. In 1978, Fujita and NCAR researcher James Wilson recorded the first microburst observed on radar.
Initially, Fujita’s theories countered conventional wisdom; other meteorologists believed downdrafts weakened by the time they hit the ground and posed little hazard.
Yet microbursts continued to take their toll. On Aug. 2, 1985, Delta Air Lines Flight 191 crashed on approach to Dallas-Fort Worth International Airport in Texas (U.S.). The accident killed 137 people and spurred further research on microbursts and wind shear.
Eventually, Fujita’s work led to the installation of terminal Doppler weather radar (TDWR) at major airports. Also, airline pilots began to receive mandatory training on microbursts and wind shear.
That work started paying dividends. On a single day — July 11, 1988 — four successive United Airlines flights encountered microbursts on approach to Denver Stapleton Airport in Colorado (U.S.). Each crew flew a missed approach and then landed safety. A fifth aircraft flew a missed approach without entering a microburst. None of the aircraft were damaged, and no passengers were hurt.
Microbursts have an especially sinister trait: On first encounter, they can lure fliers into danger. When a downdraft strikes the ground, winds flow up and out from the impact point. An aircraft approaching this downdraft initially experiences a head wind that causes higher indicated airspeed. This increased performance can tempt a pilot to reduce power. But if the pilot does that, the engines are spooled back as the aircraft flies into the main column of descending air. Airspeed drops and the head wind shifts to a tail wind. Now the aircraft becomes vulnerable to a stall or to powerful downdrafts pushing it toward the ground.
Fortunately, microbursts don’t last long — typically no more than 15 minutes. Pilots can often avoid them with a brief hold.
Researchers and engineers realized an on-board wind shear detection system could help pilots recognize that trap. In 1987, the FAA first proposed requiring airborne wind shear detection equipment on airliners.
Current U.S. rules require on-board wind shear detection on jet airliners, and other regulators, including the European Union Aviation Safety Agency, have similar requirements.
Some aircraft have both predictive and reactive wind shear warning systems. The predictive function alerts pilots to wind shear conditions ahead, and the reactive function alerts when wind shear is entered. Both systems work by measuring vertical and horizontal wind speeds. During a wind shear event, the systems typically provide an aural “wind shear” warning and a visual indication on the primary flight display.
Wind shear escape procedures may vary somewhat, depending on the airline and the aircraft model. But generally, the guidance calls for an immediate go-around with maximum thrust, while maintaining landing gear and flap configuration until out of wind shear. During approaches when wind shear is anticipated, pilots normally increase airspeed up to 15 kts. The added speed provides a wider stall margin, and greater kinetic energy in the event of a missed approach.
Flying wind shear escapes requires simulator practice. When an aircraft emerges from wind shear at full thrust, pilots can easily exceed the published missed approach altitude if that altitude is fairly low, such as 3,000 ft. Pilots can also exceed the 250-kt speed limitation below 10,000 ft. But with training, they can learn to reduce power and pitch down at the right moment. Teamwork is essential — when the pilot monitoring sees vertical speed increasing and calls “out of wind shear,” that helps the pilot flying know when to pull back the thrust levers.
Wind shear detection technology continues to improve. Some of the latest ground-based systems use LIDAR (which stands for light detection and ranging, or laser detection and ranging). The system works by emitting infrared light into the atmosphere and measuring beams reflected by particles in the air.
Sydney Airport in Australia is among the airports that have recently installed LIDAR.
This progress in technology and training has made the skies safer. A 2022 paper prepared for the American Meteorological Society noted that there had not been a commercial microburst-related accident in the United States since the July 2, 1994, crash of a USAir Douglas DC-9 in Charlotte, North Carolina, which killed 37 people in the airplane.
The paper characterized the refinements in wind shear detection and avoidance as “one of the most successful and societally impactful [research to operations] programs in atmospheric science history.”
Image: by-studio/shutterstock
Thomas W. Young is a retired airline captain and a former instructor flight engineer with the West Virginia Air National Guard. Young has logged nearly 12,000 hours of pilot and flight engineer time.