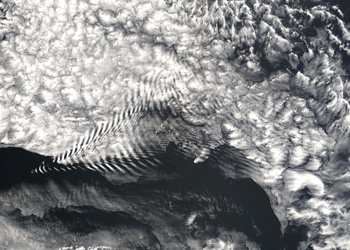
On March 5, 1966, British Overseas Airways Corp. (BOAC) Flight 911, a Boeing 707, departed from Tokyo’s Haneda Airport for Hong Kong Kai Tak International Airport. In clear skies, the flight crew attempted to fly over Mount Fuji (approximate elevation 12,400 ft). But with strong winds and an approach to the mountain from the leeside, the airplane encountered a particularly severe mountain wave over Gotemba City at 16,000 ft. The turbulence encounter was so extreme that the aircraft experienced in-flight structural breakup. All 124 people aboard were killed.
Even though today’s jetliners benefit from newer designs to withstand turbulence, pilots still are expected to avoid conditions where severe or extreme turbulence is possible. And although crashes primarily caused by turbulence have become rarer since the Flight 911 accident, they still occur, and serious incidents are common. The U.S. National Transportation Safety Board (NTSB) estimates that nearly three-quarters of all weather-related accidents/incidents are due to turbulence. The U.S. Federal Aviation Administration (FAA) reports that dozens of airline passengers have been injured in recent years by turbulence encounters. Turbulence is the number one cause of injuries to passengers — and especially to flight attendants — in nonfatal accidents. In a typical year, U.S. pilot reports (PIREPS) of moderate or greater turbulence average 65,000, and reports of severe or greater turbulence average 5,500.1
What do we mean by turbulence? In terms of airflow, meteorologists focus on laminar flow and turbulent flow. Laminar flow is a flat, regular, smooth flow of air that moves in the same direction at the same speed. Turbulent flow is chaotic, with air moving in random directions and at varying speeds. In the real world, we see both occurring at the same time. When the turbulence becomes more dominant, then there are problems. In terms of aerodynamics, turbulence is a sudden change in airflow that abruptly affects the altitude and/or attitude of the aircraft.
For this article, we are concerned only with the vertical variations in airflow. The term turbulence often also is used in conjunction with rapid variations in horizontal wind speed — that is, wind gusts. Also, by limiting this article to naturally occurring turbulence, we will not address similar problems such as wake turbulence, jet engine blast or helicopter rotor downwash.
Refresher on Basics
Turbulence can occur anywhere in the atmosphere, from ground level to near the upper limits of standard high-level en route charts, typically above Flight Level (FL) 350 (approximately 35,000 ft). To give pilots a better idea of what they are dealing with, turbulence is described in terms of intensity for reporting and forecasting. Light turbulence causes just brief and slight changes in altitude and/or attitude. Moderate turbulence is of greater intensity and also may cause changes in airspeed, but the aircraft remains in control at all times. Severe turbulence can cause large, abrupt changes in altitude and/or attitude and can cause large changes in airspeed. The aircraft may be temporarily out of control. With extreme turbulence, an aircraft can be tossed about violently and may incur structural damage.
In the United States, pilots are urged, in the common interest of safety, to report encounters with moderate or greater turbulence to air traffic control (ATC) in a PIREP. According to FAA guidance, ATC expects turbulence reports to include location, altitude or range of altitudes, and aircraft type, and should include whether the aircraft was operating in clouds or clear air. In this system, the reported turbulence intensity (light, moderate, severe or extreme) and duration (occasional, intermittent or continuous) are determined subjectively by the pilot (see “Smooth Operators”).
Turbulence can be broken down into three major categories by cause: mechanical, convective and wind shear.
Mechanical turbulence occurs when airflow encounters a physical impediment (Figure 1). The air flows around, over and, if the object is off the ground, under the obstacle. But when the air is forced around an object, the flow often becomes more turbulent. Waves and even vortexes can form downwind of the object. Depending on the wind direction and speed, buildings at an airport, for example, can cause turbulence that can affect aircraft takeoff/landing performance on a runway, or — if surface winds exceed 50 kt — flight at altitudes greater than 3,000 ft above ground level (AGL).
Mountain waves, such as the one that destroyed Flight 911, fall into the category of mechanical turbulence. On the windward side of a mountain, the air is forced upward. It will then sink on the leeside. Often vertical waves are formed. These waves can break like ocean waves and generate significant turbulence. Although single mountains can produce waves, mountain ranges have greater effects on airflow and are more prone to significant mountain waves. The waves can propagate a great distance from the mountain source, up to 100 mi (160 km) or more downwind. At times, a set, or train, of waves is established in the lee of the mountain. And the induced waves can extend higher than the mountains that produced them. Sometimes these waves are marked by visually striking cloud formations, such as lenticular clouds or roll clouds. At other times, the air may be deceptively clear.
Convective turbulence can be as innocuous as a faint updraft on a warm day or as potentially destructive as the violent updrafts and downdrafts in a thunderstorm. For many operational purposes, convection — caused by vertical currents (thermals) that develop in air heated by a warm surface below — can be simply considered warm air rising and cool air sinking. Glider pilots, for example, know where to find the rising thermals that help keep their aircraft aloft. Cumulus clouds are convectively produced. Airliner occupants may experience a bumpy ride from the minor vertical motions, even through a small “fair weather” cumulus cloud, but prolonged flight in these conditions would be considered an undesirable ride-quality event.
Frontal zones, where two differing air masses collide, are frequent breeding grounds for turbulence, so much so that “frontal turbulence” is often included as a separate category to emphasize its importance. With colder air being denser, the warmer air is forced up over it, often producing vertical waves and vortexes that generate turbulence. Fast moving cold fronts are usually the most turbulent.
The turbulence within thunderstorms is well documented. Professional pilots, even those operating large commercial jets, know to avoid the associated turbulence. The updrafts and downdrafts in the strongest thunderstorms can exceed 100 mph (160 kph). The turbulence generated can structurally damage even the strongest aircraft. Don’t be misled by the “hurricane hunter” missions you hear about. The tropical convection, even in a powerful tropical cyclone, isn’t nearly as strong as in midlatitude thunderstorms.
Disturbingly, there still are numerous accidents with pilots miscalculating the danger of the destructive turbulence within these storms. For example, on March 25, 2010, the experienced pilot of a Eurocopter AS350 B3 on an emergency medical services flight, attempted to outrun an approaching line of thunderstorms and return to base in Brownsville, Tennessee, U.S. The squall line, moving at 61 kt groundspeed, apparently overtook the helicopter. The aircraft crashed in an open field 2.5 mi (4.0 km) from its destination, killing the pilot and two nurses aboard. NTSB investigators looked at weather data and concluded “the helicopter likely encountered severe turbulence from which there was no possibility of recovery, particularly at low level” (ASW, 3/12).
Turbulence caused by thunderstorms is not restricted to the area within the cloud itself. Strong downdrafts can extend below the cloud base and often reach the ground, where the winds become horizontal, creating a threat of wind shear. These rapid changes in wind speed and direction were associated with a number of major aircraft accidents in the 1960s and 1970s. With widely used automated detection of wind shear and escape-maneuver education, pilots and ATC personnel should now be well aware of the risks, and wind shear–monitoring equipment such as Doppler radar and lidar (light detection and ranging) have become standard at many larger airports.
Dangerous turbulence also can occur above thunderstorm clouds. United Airlines Flight 967, a Boeing 777-200, was en route from Washington to Los Angeles on July 20, 2010. Flying over an area of developing thunderstorms in Kansas, the plane hit severe turbulence while cruising at 38,000 ft, seemingly well above the storms. The flight crew landed the airplane in Denver, where 21 people were taken to hospitals for their injuries. Meteorologists who studied the event believe a gravity wave (ASW, 2/10) generated by the storms may have been the cause of the turbulence.
Jet Stream Factors
Although not as severe as thunderstorm turbulence or strong mountain waves, conditions known as clear air turbulence (CAT) pose a much greater threat for air travelers. Typically, these encounters occur above 15,000 ft AGL. As the name implies, CAT usually is not associated with cumuliform clouds and is, thus, unable to be detected in advance using the pilots’ eyesight. It doesn’t show up on conventional on-board weather radar. Often, it isn’t forecast. And the worst incidents associated with CAT have occurred at FL 300 or higher. Of all turbulence-related incidents, two-thirds occur at or above this level. This is a typical cruising altitude for longer flights and often a flight phase when the seat belt signs are not illuminated, and where the passengers and cabin crew are not wearing seat belts and may well be moving around the cabin. Technically, CAT also can be present in nonconvective clouds.
A major cause of turbulence at these upper levels is the jet stream. This fast-moving river of air often is concentrated between 30,000 and 35,000 ft above sea level. Wind speeds in the core of the jet stream can exceed 200 mph (320 kph) and may approach 300 mph (480 kph) in some cases. The turbulence in these situations is generated by the wind itself, and is a result of wind shear.
For flight operations, we often think of wind shear as a low-level phenomenon. Often in that case, it’s a quick, unexpected change in wind speed and/or direction associated with a thunderstorm downdraft. Technically though, wind shear is the change in the vertical component of wind speed or wind direction.
A jet stream, however, is a three-dimensional weather system. It’s not just a flat ribbon of strong winds at one level in the atmosphere. It has a vertical extent, and strong winds may reach down thousands of feet below the jet stream core. But as you move away from the core of the jet, the wind speeds decrease. This sets up the wind shear. Once again, the jet’s flow can become turbulent, with waves and even vertical vortexes. These can contain the abrupt vertical motions that induce the turbulence that can affect aircraft even some distance away from the jet itself. In fact, the worst turbulence isn’t within the core of the jet stream but rather away from the core, so that is where the wind shear is strongest. And this can occur both above and below the jet core and to the sides of it.
One of the worst cases of jet stream turbulence effects occurred Dec. 28, 1997, when United Airlines Flight 826, a Boeing 747-122, en route from Tokyo to Honolulu encountered severe CAT over the Pacific Ocean about 870 nm (1,610 km) east-southeast of Tokyo. One passenger was killed and 171 passengers were injured, 15 seriously. Nine crewmembers also were injured, three seriously. The plane plummeted 1,000 ft when it encountered the turbulence at FL 330. The woman killed wasn’t wearing a seat belt and apparently was thrown against the ceiling. (Technically, the ceiling hit her.) The flight crew returned to Tokyo and made an uneventful landing. The accident pilot had reported visual meteorological conditions with no clouds. Turbulence had not been forecast. A 140-kt jet stream was in the area with its core centered near 39,000 ft.
There are two major jet streams at upper levels of the atmosphere above Earth’s Northern Hemisphere — the polar jet and the subtropical jet. The polar jet is tied in with the polar front, the dividing line between warm, tropical air and cold, polar air. Seasonally, the polar jet moves well north in summer, often poleward of 45 degrees latitude. In the winter, it follows the cold air toward the equator. It can dip down as far as 25 degrees latitude.
The polar jet is highly variable in both location and strength. It can move far down into midlatitudes with outbreaks of polar and even arctic air. But it can also surge back poleward as warm air pushes up ahead of major storm systems. Although typically found near 30,000 ft, the core of the polar jet can drop to 25,000 ft or even lower.
The subtropical jet, often centered near 35,000 ft, is found at lower latitudes, usually between 20 degrees and 30 degrees. It typically affects the southern United States and Mexico, the Mediterranean region, and Southeast Asia up to Japan. Both jets are much stronger and more important to aviation in the winter.
Often the jet stream is depicted as a solid arrow following the airflow above the ground below. This gives the impression that jet stream winds are continuously strong along the jet axis (the drawn arrow). They are not. Wind speeds vary greatly along the jet axis with areas of weaker winds separating areas of stronger winds. The areas of stronger winds are called “jet streaks.” This is where temperature contrasts at upper levels are strongest, the so-called “upper-level fronts.” These streaks would be associated with the strongest wind shear and maximum turbulence. The jet streaks themselves also move or propagate along the jet axis. But they move at much slower speeds than the winds themselves, usually at 20 to 40 kt. Closer to Earth’s surface, low-level jets (ASW, 11/12) could also produce turbulence.
To forecast turbulence in the United States, the online Aviation Weather Center of the National Weather Service provides turbulence products for aviation. For example, all PIREPS containing turbulence data are displayed on a map. SIGMETs (significant meteorological information) for turbulence and convection are available. There are also maps showing areas forecast to have turbulence. One of the center’s latest developments is called Graphical Turbulence Guidance, an automatically generated turbulence product that predicts the location and intensity of turbulence over the continental United States.
In the future, will global warming affect turbulence? In an article published in Nature Climate Change, researchers from the University of Reading in England say that it will.2 Looking specifically at air routes over the North Atlantic, they predict that winds above 10 km (33,000 ft) from Earth’s surface will strengthen. This could lead to a 10 percent increase in events involving moderate or greater turbulence, they said.
Edward Brotak, Ph.D., retired in 2007 after 25 years as a professor and program director in the Department of Atmospheric Sciences at the University of North Carolina, Asheville.
Notes
- U.S. National Center for Atmospheric Research (NCAR), Research Applications Laboratory. Aviation Turbulence.
- See <www.bbc.co.uk/news/science-environment-22063340>. Accessed March 16, 2017.