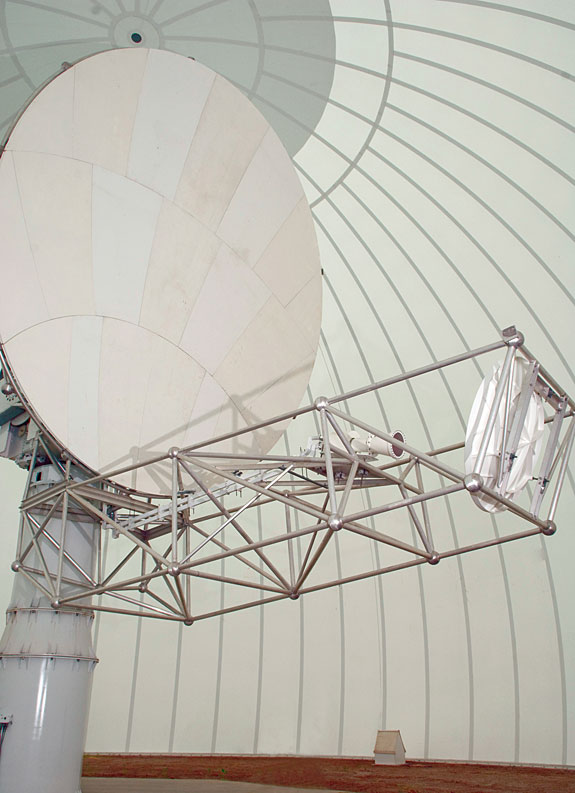
Radar has become an indispensable tool for weather forecasting and plays a critical role in aviation safety. Simply put, weather radar is the best tool that meteorologists have to predict general precipitation and to develop short-term forecasts of severe weather.
A weather radar display provides a comprehensive picture of the weather in real time. For aviation interests, radar provides a depiction of weather hazards such as thunderstorms in detail unavailable from any other source.
Radar and aviation go way back. The term radar is an acronym derived from radio detection and ranging, the original name of a technology that initially was based on the use of radio waves. Radar was developed to track aircraft in flight and was first widely used during World War II.
During the war, radar operators noticed that their equipment detected not only aircraft but also areas of precipitation. This presented a problem because the display of enemy airplanes could be lost in the “clutter” that resulted when the radio waves were reflected by raindrops. However, meteorologists looked at this “problem” differently and foresaw radar as a tool for detecting precipitation. By 1943, the military was successfully using radar to monitor areas of rain and to make short-range weather forecasts.
After the war, experimentation with surplus military radar equipment was begun to determine if radar could be useful in the burgeoning science of weather forecasting. In the late 1950s, the Weather Surveillance Radar System was set up in the United States with approximately 60 sites. The radar equipment was updated, and more sites were added in the 1970s and again in the 1990s.
Today, more than 150 radar sites operated by the U.S. National Weather Service (NWS) provide radar coverage for most of the country.
Echo Location
The principle of radar is simple. A pulse of electromagnetic energy — microwaves are used today — is transmitted from the radar site. The radar transmitter does a 360-degree sweep while emitting microwaves at a constant elevation angle (usually 0.5 degree). When a portion of the energy hits an object, it is reflected back to the radar antenna. How much energy comes back and from where are recorded. The object’s distance from the radar site can be determined by measuring the time it takes for the energy to be transmitted and to return to the antenna.
The reflected energy is reproduced electronically on the radar screen to show the object’s relative position and distance. The displayed object is called a radar return, or more commonly an echo. The image shown on a standard radar display is called the base reflectivity.
Radar is capable of detecting precipitation more than 200 mi (322 km) away. Raindrops are excellent reflectors of electromagnetic energy. The larger the raindrop, the greater the reflection. Larger raindrops are associated with higher or more intense rainfall rates. So, in addition to position and display, the intensity of the precipitation can be depicted.
Color Coding
On the old monochrome radar screens, higher precipitation intensities would show up simply as brighter echoes. As radar technology advanced and the displays featured higher resolutions, the echoes were colorized to make differences in precipitation intensity more obvious.
The colors seen on a standard radar display today correspond specifically to the returned energy, technically as measured in dBZ, or decibels of Z (a radar term). The range of colors and their associated dBZ levels typically are shown in a legend on the side of the display.
For example, is the base reflectivity image recorded by the radar site in Sterling, Virginia, at 0248 coordinated universal time on June 30, 2012. Clearly depicted is a wavy line of red echoes with a maximum reflectivity of 62 dBZ. This is the extremely powerful derecho squall line system that moved through the area that evening. Winds at Washington Dulles International Airport gusted to 62 kt.
For practical purposes, the display colors correspond to rainfall rates and to storm intensity. Blues and greens indicate light rain. Sometimes, although it shows up on radar, this rain does not even reach the ground and is known as virga. Yellow, orange and red correspond to heavier rainfall, likely associated with convective activity.
Snow can be detected by radar, but it does not show up as well as rain. The crystal structure of snowflakes does not provide a good reflective surface. And snow has lower water content. The old rule of thumb that 10 in (25 cm) of snow equals 1.0 in (2.5 cm) of water means that a heavy snow equates to a light to moderate rainfall.
The radar itself cannot discern the difference between rain and snow. The radar displays often seen on television, which show snow in a different color, have been augmented with additional information.
Clouds are not detected by radar. The droplets or ice crystals that make up clouds are too small to reflect the microwave energy. Interestingly, radar can detect flocks of birds or bats, and at times even swarms of insects, which are all better reflectors than clouds.
Using radar equipment for forecasting is a fairly straightforward process. Meteorologists observe an area of precipitation over time and determine its speed, direction of movement and any changes in intensity. (Precipitation seldom simply develops over any particular region. Usually, it moves into a region.) Once the speed, direction and intensity trend are known, a continuity forecast can be made by projecting further movement of the precipitation area.
Meteorologists can use this process to forecast precipitation for the next several hours. Short-term forecasts for severe storms actually can be done by computers linked to the radar equipment.
Cones and Clutter
There are some problems and limitations in using radar for short-term forecasting. Precipitation directly above the radar site and in the immediate surroundings cannot be detected; the radar beam does not travel upward through this so-called cone of silence.
Microwaves cannot penetrate solid objects; thus, we have the problem of ground clutter. Nearby buildings and even distant mountains reflect the electromagnetic energy. The resulting false echoes can be deleted automatically from the radar display, but any real precipitation behind the clutter does not show up on the screen. To allow for this, the radar transmitter is tilted slightly upward. This creates its own problem, however, because the radar beam can overshoot areas of precipitation that are far away.
Because of the tilting of the transmitter, as well as the curvature of the earth, the maximum range of precipitation detection is about 100 mi (161 km) for most precipitation and 200 mi (322 km) for intense precipitation.
Anomalous propagation is another effect that can produce false echoes. Certain atmospheric conditions, such as temperature inversions (warmer air overrunning colder air), can cause the transmitted microwaves to bend or refract, rather than move in straight lines. Similar to a mirage, surface objects can appear on the radar screen to be airborne. This situation often develops at night and explains the false echo area that engulfs the radar site.
Storm Detection
Thunderstorms always have posed a tremendous threat to aviation. As mentioned earlier, the idea that radar could be used to detect and track thunderstorms was recognized almost immediately by the aviation community. In the late 1940s, in addition to the establishment of ground-based sites, on-board radar equipment began showing up in aircraft. By 1964, all passenger airplanes in the United States were required to have on-board radar systems. Today, weather radar equipment also is standard at major air traffic terminals.
Interestingly, radar cannot directly tell where thunderstorms are occurring. It cannot detect electrical activity (although some modern on-board systems now have “lightning-detection” capability). Nevertheless, rainfall rates in thunderstorms usually are extreme; therefore, it can be inferred that precipitation cells with very heavy rainfall rates also may contain lightning and, more importantly, extreme turbulence.
Most standard radar displays use red to indicate echoes that likely came from a thunderstorm. Also, if there is a possibility that thunderstorms are within range, the horizontal sweep can be stopped, and the radar can be aimed at the potential thunderstorm cell to scan it vertically. This provides cloud top information that can be used to determine possible electrical activity and turbulence. The higher the cloud top, the greater the likelihood of lightning and strong turbulence. Cloud top information also can be used by pilots to determine if flying over the storm is feasible.
Hail produced by thunderstorms poses a tremendous threat of damage to aircraft. It also is indicative of extreme updrafts and strong turbulence. Hail has a very high reflectivity. A dBZ value over 60, which shows up as purple on the display, indicates large hail.
Bow echo squall lines frequently produce the strong winds that can generate the extreme low-level wind shear that is so dangerous to aircraft taking off and landing. The phenomena of bow echoes were discovered through radar analysis. As was determined, whenever a line of thunderstorms curves, or bows out, there likely are strong winds in the bowing part of the line.
The system that affected the Washington area displayed the bowing characteristic. In fact, this system had developed 12 hours earlier in Illinois and had moved southeastward during the day, continuously producing bow echo thunderstorm lines and associated strong winds. Radar clearly showed this, and advanced warnings certainly prevented more casualties from occurring.
Among other aviation hazards that can be detected by radar are pulse thunderstorms, which can quickly produce strong downdrafts and dangerous wind shear (ASW, 10/09, p. 12). Warnings can be issued when the characteristic vertical radar profile of a pulse storm is detected.
At times, the outflow boundaries, or gust fronts, from convective weather systems — which also can contain strong low-level winds and wind shear — shows up on radar.
The strongest of all thunderstorms, the supercell, also has a distinct radar image. The base reflectivity image produced by the Oklahoma City radar site the evening of May 3, 1999 shows this massive supercell thunderstorm had a telltale appendage, the classic hook echo, indicative of strong winds, large hail and, in this case, a devastating F5 tornado.1
Doppler Effect
Doppler is a term we often hear today when describing radar sites or radar displays. It can be misleading. The Doppler component is just one of many capacities that most new radar sets have. It has nothing to do with the detection and tracking of precipitation. True Doppler displays seldom are used.
Doppler’s primary purpose is the detection of potentially severe thunderstorms. The Doppler effect, or Doppler shift, is the change in frequency of a wave when there is movement between the wave emitter and receiver.2 The classic example is the change in pitch of a train whistle as it approaches and then passes the station. The sound waves are impacted by the movement of the train relative to the listener.
In radar application, the microwaves reflecting off a moving target change frequency as they come back to the radar site. This change in frequency can be measured and is proportional to how fast the target is moving toward or away from the radar receiver. Simply stated, Doppler shows which way the air is moving relative to the radar site and how fast it is moving.
Color is used to make the Doppler display easier to interpret. Winds blowing toward the radar site are depicted with “cool” colors such as green and blue, and are given negative values. “Hot” colors such as yellow and red indicate air moving away from the site and are shown as positive values.
Tthe base velocity Doppler product from the Sterling radar site shows as the squall line passed over the site, strong winds from the west-northwest were blowing toward the site and away from the site to the east. The strongest depicted velocities were in the 60- to 70-kt range.
In addition to the depiction of strong winds, Doppler can be used in other ways to detect severe thunderstorms. Rotation is a characteristic of most strong to severe thunderstorms. Doppler can detect such rotation from “couplets” of airstreams moving toward and away from the site in close proximity. This is depicted on the radar screen as a hot color next to a cold color. The faster the air is moving, the stronger the rotation and the more likely the storm will be strong to severe.
The Doppler image from the Oklahoma City storm shows the blue pixels next to the orange pixels indicate its intense rotation.
Relative velocities recorded from previous storms are stored in the radar site’s computer and used for comparison with current readings. When certain storm elements such as a mesocyclone or tornado vortex signature are detected, automatic warnings are generated. This is the origin of the now familiar phrase “Doppler-indicated” severe weather. Meteorologists no longer have to wait for severe weather to actually be observed before warnings are issued.
Doppler radar presented another major breakthrough for aviation. Back in the 1970s and 1980s, a number of fatal aircraft accidents were caused by wind shear associated with intense thunderstorm downdrafts or microbursts. Doppler radar usually can detect this type of wind shear, but only if it is fairly close to the radar site. Microbursts can be detected out to about 20 mi (32 km).
The NWS Doppler radar network established in the late 1980s and early 1990s left many areas without coverage. As mentioned, however, a number of major terminals have installed their own Doppler radars. Today, there are 45 terminal Doppler weather radar sites in the United States, and many aircraft are equipped with on-board Doppler radar wind shear sensors.
An important consideration mentioned in the July issue of ASW (“Weather Warning”) is to always check the time on a display of radar information. In particular, mosaic images derived from multiple Next Generation Radar (NEXRAD) sites may be as much as 20 minutes older than indicated on the display. This can be especially crucial for convective situations in which thunderstorms move at speeds of 50 mph.
Edward Brotak, Ph.D., retired in 2007 after 25 years as a professor and program director in the Department of Atmospheric Sciences at the University of North Carolina, Asheville.
Notes
- F5 is the highest rating on the Fujita-Pearson Scale, indicating wind velocities greater than 261 mph and capable of causing “incredible damage.”
- The effect was hypothesized in 1842 by Austrian physicist Christian Doppler.