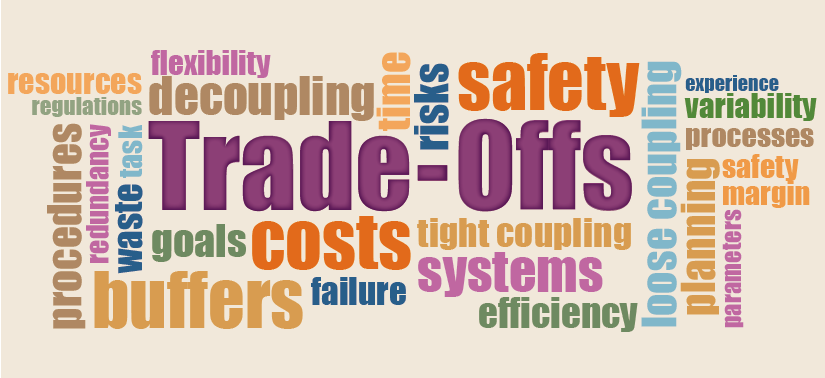
“Given the ATIS (automatic terminal information service) other conditions and our drive to operate as efficiently as possible, we [the flight crew] planned for takeoff from runway beginning and [to] have the full runway available, … [which] allows us to use maximum engine thrust derate, which is cheaper in fuel and engine wear.
“Fifteen minutes behind our departure schedule, we started the pushback … with many passengers that had onward connections at our destination. Initially, we lost time because we were missing a passenger, so luggage had to be offloaded. Next, we had an aircraft [stopped] behind us [on the ramp] with some technical problems, which caused a further delay of seven minutes before we could finally push back.
“We were approaching the runway, and we had one aircraft ahead of us. Our senior purser reported ‘cabin ready’ shortly before we arrived at the runway holding area. Approaching the runway, the ‘before takeoff’ checklist was completed. The aircraft ahead of us reported an issue and informed [the control] tower they needed maybe three more minutes. We were not happy to encounter more delay. Delaying the takeoff could also bring thunderstorms, approaching from the west, into play. We had to choose: Wait behind the other [aircraft] and be further delayed with weather moving in, or ask the tower if we could take an intersection [takeoff].
“The tower cleared us to taxi and line up at the runway intersection, which gave us 2,950 m [9,679 ft] of takeoff runway instead of 3,100 m [10,171 ft]. We realized we had to update our full runway takeoff performance calculation to an intersection takeoff; the intersection was selected, [and the] assumed temperature was lower than the original, which was what we expected. Updated speeds were inserted in the flight management system while the tower cleared us for takeoff. We even adjusted the trim, and that gave a nice feeling of being ‘right on the ball.’ We lined up and selected takeoff thrust. While the engines were spooling up, my colleague suddenly called, ‘Oh! The flaps!” We made a low speed rejected takeoff. ”
This real-life experience narrated by Arthur Dijkstra, a Boeing 777 captain and a safety researcher, is a useful example of the considerable number of “couplings” in air operations. Couplings are dependencies among different tasks of an operation.
The challenge for pilots often is minimizing costs without compromising safety, says Dijkstra. “You plan for a specific situation and try to be as efficient as possible,” he says. “You try not to lose time and you try to reduce costs as much as possible by [using] maximum derated takeoff thrust. … While constantly monitoring the requirements for a safe flight execution, we pride ourselves in minimizing costs. The aviation and airline system is not like a clock that ticks precisely in a fully controlled, closed environment. Because of external disturbances or technical issues, people operating these systems constantly need to make adjustments to make the system work. We try to work as efficiently as possible in a complex world.”
The effect of couplings in complex production systems was described by Yale University sociologist Charles Perrow in his book, Normal Accidents, which was published in 1984. The book was written in the aftermath of the Three Mile Island nuclear reactor accident near Middletown, Pennsylvania, U.S., in March 1979. Perrow developed, or at least widely disseminated, the notion of tight vs. loose coupling in modern production systems, and also addressed the issue of complexity vs. linearity.
According to Perrow, tight coupling is “a mechanical term meaning that there is no slack or buffer or give between two items. What happens in one directly affects what happens in the other.” On the other end of the spectrum, as Perrow explains, is loose coupling, where performance standards are ambiguous or flexible. In loose coupling conditions, slack (surplus) resources are available to meet unexpected challenges.
Perrow further defines the tight-loose coupling dichotomy in accordance with a series of features: time dependency, variability of time sequences and process design, slack, and recovery from failure. Tight coupling is characterized by time-dependent processes that cannot wait to be completed beyond a narrowly determined time frame. In conditions of loose coupling, delays are possible and processes can remain in stand-by mode if necessary.
The timing of processes designed to work in conditions of tight coupling are more invariant than in loose coupling. In conditions of tight coupling, there is only one way to make a product or deliver a service, so it follows that a given task must be followed by another and so on. Also, quantities must be precise, resources cannot be substituted, wasted supplies overload a process, and failed equipment entails discontinuing the operations. Buffers, redundancies and substitutions need to be planned for, and designed into, the process.
In a loose coupling situation, tasks can be re-routed and supplies, equipment and manpower can be wasted without great impact on safety performance. A process can be repeated if it is not done correctly the first time. Expedient, spur-of-the-moment buffers and redundancies and substitutions can be found without advance planning.
Tightly Coupled Aviation System
Tight coupling is a defining feature of commercial aviation as a system and is closely associated with efficiency, a property that Dijkstra defines as being “about the resources needed to achieve a goal or to execute a process. When a work environment is ordered, stable and predictable, free of unexpected disturbances, and the goals do not change, we can focus on efficiency. By process optimization, costs can be reduced by eliminating waiting time, fuel burn, peak loads, variability, etc. These factors can be seen as waste and need to be reduced to improve efficiency. An efficient process achieves exactly its specific goals in a specific predefined situation.”
There are a variety of areas within aviation where coupling places considerable pressure on the performance of human operators and supports a very efficient utilization of aviation assets.
Air traffic management procedures, for example, show several levels of coupling, depending on the flight phase. “The closer the aircraft is to the airport area, the tighter the connections. En route, aircraft usually have longer separations in terms of time and distance; so this by default allows for buffers in order to tackle in-flight emergencies, make decisions, instruct air crews, etc.,” says Nektarios Karanikas, a safety management professional and scholar. “During the approach and landing phases, where the air traffic is denser and the temporal factor plays a paramount role, time-dependency is more visible; however, in case of developing emergencies, there is some space for devising alternative ways for accomplishing the high level goal (i.e., safe landing of the aircraft).”
Aircraft ground handling is another tightly coupled domain. “The emergence of low-cost carriers, and the continuous effort of aerodromes to increase their capacity under lower costs, has led to tightly coupled procedures, many of which are performed in parallel with others,” says Karanikas. “Refueling, luggage off-loading and loading, catering services, aircraft inspections and flight line service need to be accomplished in time, most of them concurrently, and with the least possible resources. Also, the current regulatory framework does not allow flexibility in the design of some processes, due to restrictions imposed for safety reasons (e.g., when refueling the aircraft, the boarding of passengers is not typically allowed, except in rare cases where a waiver is issued),” says Karanikas.
On the flight deck, checklists are tightly coupled systems, according to researchers Degani and Wiener. Checklist procedures are coupled to other tasks, such as starting engines, takeoff and landing. A checklist can be coupled by design, such as a takeoff checklist that must be accomplished on the active runway or just prior to entry onto the runway. When a checklist is tightly coupled, the buffers embedded in the system (redundancies and backups) are bypassed, and the ability of the crew to recover from a failure is diminished (ASW, 12-14/1-15).1
“There are some checklists that cannot be decoupled,” says Marcel Martineau, a former long-haul pilot with Air Canada and now a flight operations consultant, citing as examples the takeoff and landing checklists, the latter of which includes items such as extending the landing gear, flap settings. “There are several other checklists that are tightly coupled, such as engine failure on takeoff, depressurization, go-around procedures. In fact, they are so tightly coupled that you have to learn these procedures by heart as you do not even have time to refer to a checklist,” Martineau says.
Buffers
Tight coupling is not inherently good or bad, it simply characterizes the way many procedures are accomplished in the aviation work environment. However, the decoupling of work processes, if possible and practicable, may improve safety performance because of the redundancies introduced by buffers.
“If we look at the network of flight operations, we can easily appreciate to what extent we have to deal with complexity in aviation. Other traffic and ATC [air traffic control] decisions influence us and we have to adapt,” says Dijkstra. “One of the features of complexity is the uncertainty about the situations we are going to find ourselves in. We need resources such as time, fuel and information to adapt to these unexpected situations. These resources also work as buffers to protect us against immediate effects from changes in our environment. The buffers can absorb disturbances — at least in part and for a certain period of time — and give us time to think, act and check their effects on our goals. A rain shower over our destination can affect us in different degrees, depending on the fuel we have on board and the slack we have in our scheduled time of arrival.
“Some degree of buffers and resources to handle the unexpected is designed into the system. We always have fuel [to reach an alternate destination], which would not be necessary if the world were fully predictable. We train more skills than the minimum required, which supports us in handling all kinds of situations,” he says.
Practical decoupling of procedures can be accomplished by adding buffers such as “reviewing the takeoff parameters while at the ramp before engine start,” says Martineau. “This can be performed early at the gate and just confirm that the takeoff weight is within certain parameters. The landing briefing and taxiing procedures can be reviewed at a convenient time while approaching the top of descent point.”
Degani and Wiener say that “the critical checks should be completed earlier in the ground phase in order to decouple the critical items from the takeoff segment, as well as to allow enough time (buffers) for the crew to detect and recover from a configuration failure.”2
Sometimes line employees also can create and manage buffers and margins in real-time operations. “Planning for [takeoff at the] runway intersection would have made us more effective and less sensitive to outside disturbances, such as the preceding aircraft that was not ready for departure,” says Dijkstra, referring to the takeoff scenario described at the beginning of this article. “We would have been more effective [but] less efficient and without the need to adapt. The adaptation (quick performance recalculation for the intersection takeoff) increased the risk. The drive for maximum efficiency, planning for full runway [takeoff], made us more sensitive to changes in our environment,” he says.
“Efficiency can increase operational safety risk and we have to manage this trade-off. Sometimes we encounter non-standard situations in which we have to improvise. During the improvisation, we often try to minimize loss of time. We try to be efficient. We then try to multitask, starting a new task while waiting for the other to finish, or postpone an action and remember to do it later. In such situations, we trade time as a buffer and resource for efficiency. However, situations might develop different[ly] from what we expected and [relying on] memory to do things at a later stage is untrustworthy and thus risky,” says Dijkstra.
Efficiency Trade-off
But buffers come at a cost. “It is obvious that decoupling goes along with the planning for buffers (i.e., extra time or additional resources) and creating space for flexibility and variability; however this may be a simplistic approach” says Karanikas.
The introduction of buffers may result in additional costs or lower revenue, and unless they are required by regulators, they eventually may be discarded by companies that see them as sources of waste. The rationale for the buffers must be understood and accepted, and the buffers must be incorporated into procedures and utilized regularly. “The less buffers are utilized, the less the perceived probability of using them in the future, the higher the possibility of gradually removing them from the system,” says Karanikas.
But then, a failure to respond appropriately to an unexpected event may lead again to planning for buffers. “This shapes a vicious cycle: Over time, a focus on cost reduction and successful market competition will dominate organizational decision making, and buffers will start disappearing,” Karanikas says. “Organizations might introduce policies and procedures for anticipation, monitoring and response, but they do not really learn. [Because] adverse events are rare, past experience with accidents starts playing a minor role in planning; reliance on success creates overconfidence.”
On top of the additional costs generated by adding buffers, the flexibility and variability that buffers introduce often are not acknowledged by those charged with developing procedures. “Existing standards do not explicitly recognize the interconnectivity and interdependencies of individual system components and activities,” says Karanikas. “This increases the distance between work-as-designed and work-as-done.” Employees, due to conflicting goals and priorities, may devise shortcuts that are essentially unapproved buffers. “In time, a fallacy develops: As long as outcomes are positive, employees must have followed exactly what is prescribed in the procedures; whenever an adverse event occurs, the premise is that the staff involved most probably did not adhere to the established rules,” he says.
Moving on
According to Karanikas, both regulators and organizations have a role to play. “Authorities should enforce regulations stating high level goals and not adopt a micro-management approach which constrains flexibility,” he says.
“Regulations might additionally formulate explicit requirements for planning buffers into systems and provide specific standards (e.g., percent of total costs planned for buffers per resource type); the latter measures would ensure the ability to monitor the implementation of requirements for planning buffers into systems,” says Karanikas.
Operators should engage line employees in the planning for buffers to ensure the implementation of effective and efficient practices. “Aviation enterprises should decentralize risk management by setting risk boundaries and thresholds per level and function. Continuously sharing best practices and experiences about using buffers and flexibility safely can also comprise a means to transform tacit knowledge into widely available information” says Karanikas.
According to Dijkstra, operators should also be satisfied when they make conservative, or less than maximally efficient, decisions: “The acceptance for a possible inefficiency, e.g., planning for the intersection [takeoff] and getting the full runway, is a way to simplify and be less risky. Only with hindsight can we say we were less than optimally efficient. This should not be a disappointment because being less risky and maintaining the margins is something which can also give us pride.”
Should we always plan for an intersection takeoff and then use the full runway? “The cost involved is additional engine wear. Most takeoffs are as planned. I believe the idea could be to present the option to the pilots in such a way that if the intersection takeoff is required at the last minute, less chance of error will occur with a simplified procedure. For some operations, the option of an intersection takeoff is always available” says Martineau.
“Much of our professional pride is based on being very efficient in achieving our goals, such as being safe, on time, minimum fuel, with happy passengers,” says Dijkstra. “In some situations, this satisfaction for efficiency should perhaps be replaced by our satisfaction for reduced risk. Think about how you make the trade-off and how you manage efficiency and complexity to avoid being risky.”
Mario Pierobon is a safety management consultant and content producer. He currently is working on a research project investigating aircraft ground handling safety.
Notes
- Degani, Asaf; Wiener, Earl L. Human Factors of Fight-Deck Checklists: The Normal Checklist, Contract No. NCC2-377. A report prepared for the U.S. National Aeronautics and Space Administration Ames Research Center. May 1990.
- Ibid.
Images: Susan Reed