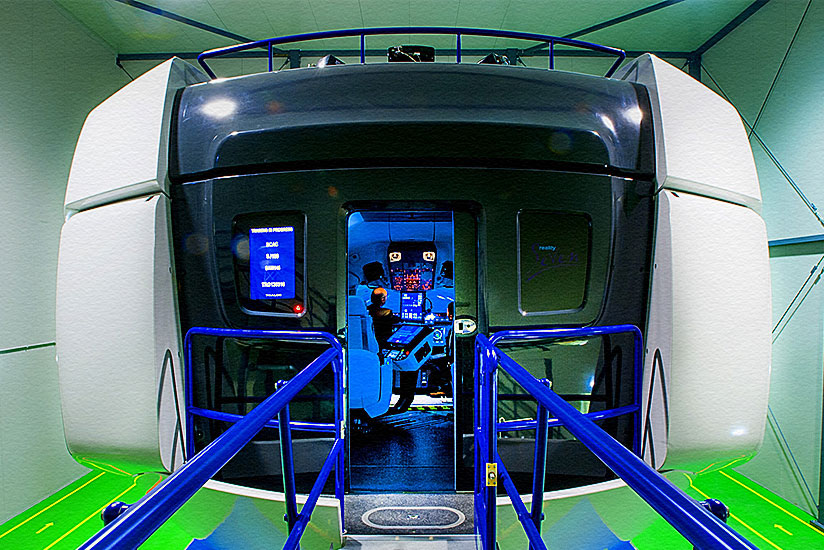
Transfer of learning is defined broadly as the ability to apply knowledge or procedures learned in one context to new contexts.1 If a habit pattern learned in one context supports an activity performed in another context, it is considered positive. However, if a habit pattern learned in one context interferes with activity performed in another context, it is negative. But habits learned in order to fly one type of airplane may not transfer to another.
For example, the first airplane the U.S. National Aeronautics and Space Administration (NASA) had me check out in was a support aircraft, a Douglas C-47 âGooney Bird.â The differences between it and the high performance Northrop T-38, in which I had over 1,200 hours as an instructor pilot, are evident with just a glance at the sleek supersonic jet trainer and the bulky tail-dragger.
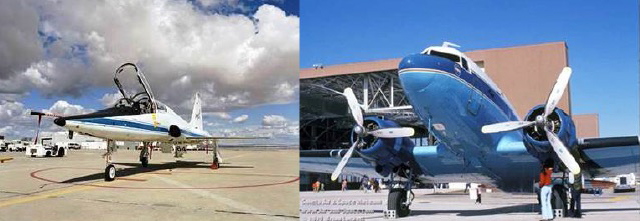
Unlike the T-38, the âGoonâ was slow to respond, and, for ground handling, required a unique coordination of brakes and power, and a lot of leg muscle. My first takeoff attempt in the C-47 was a spectacular demonstration of negative habit transfer. I careened back and forth across the runway. The oscillations increased, forcing my instructor to take control. It was grossly apparent that the habits I established in extensive jet experience did not transfer.
Had applicable simulator training existed back then, the transition could have gone more smoothly.
Advances in simulator technology mean that todayâs pilots can earn a type rating in a flight simulation training device (FSTD), formerly known as a full flight simulator (FFS) â without ever having flown the actual airplane.2 The six-degree-of-freedom3 motion base and high resolution visual displays provide a realistic training environment that transfers well to a real airplane.
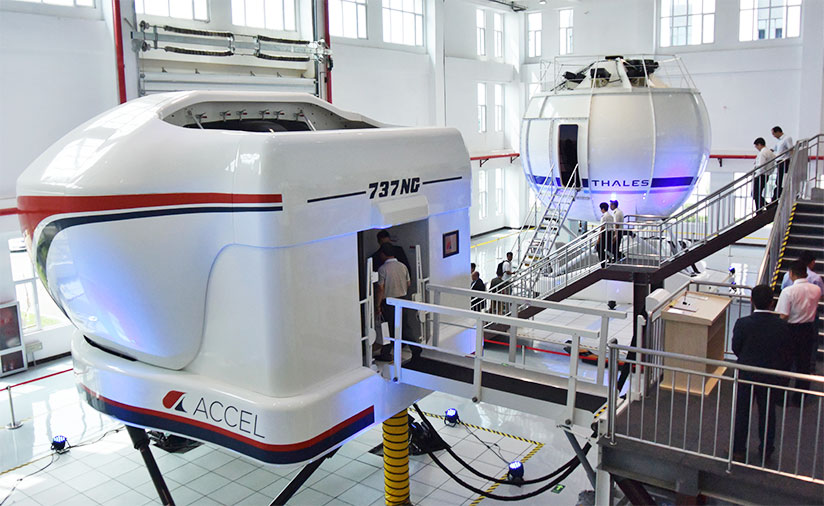
An FSTD used in flight training must have sufficient fidelity in all aspects of its form, function and operation, including a realistic emulation of motion cues to affect good habit transfer. However, because of the physical limits of an FSTDâs motion base, the ratio of inertial cues (cues from sensory organs in the inner ear that sense acceleration) to visual cues is not the same as in flight. When motion and visual cues are not congruent, pilots can become disoriented â this can even cause motion sickness in simulator training.4
New Training Requirements
Loss of controlâin flight (LOC-I) accidents represent the largest category of commercial aviation fatal accidents, and a review of worldwide transport airplane accidents from 2001 through 2010 indicated that loss of airplane state awareness has been responsible for most fatal LOC-I accidents.5
Two examples, which ultimately led to changes in pilot training and an increased reliance on flight simulators, were the 1994 crash of American Eagle Flight 4184 in Roselawn, Indiana, U.S.,6 and the 2009 crash of Colgan Air Flight 3407 in Clarence Center, New York, U.S.7 Both crashes were precipitated by a stall/upset. Although icing conditions were a factor in both cases, it was apparent that the flight crews were not prepared to recognize, prevent and recover from upsets that likely caught them by surprise. In the American Eagle crash, the surprise came from the sudden uncommanded roll from an aileron hinge moment reversal due to wing ice, and in the Colgan Air accident, from the full stall caused by disregarding the âstall pusher.â
After the American Eagle crash, a Federal Aviation Administration (FAA) special certification review team recommended that âflight crews should be exposed to training related to extreme unusual attitude recognition and recovery.â The National Transportation Safety Board (NTSB) recommended after the Colgan crash that the FAA âdefine minimum simulator model fidelity requirementsâ that support expanded stall training and recovery from fully developed stalls.
Historically, pilot type certification in flight simulators has been limited to training within the normal flight envelope. However, a law enacted in the United States in the aftermath of the Colgan crash directed the FAA to require Federal Aviation Regulations (FARs) 999Part 121 air carriers to provide stall and upset recovery training in FSTDs8 by March 12, 2019. To satisfy this new training requirement, FSTDs must meet certain high angle-of-attack modeling criteria and qualifications. Qualification of these simulators requires quantitative and subjective evaluation. Test pilots who have performed full stalls in the aircraft being simulated, or stalls in a similar aircraft, will evaluate the simulator models. FSTDs that manufacturers do not modify according to FAA requirements will be limited to use in âapproach to stallâ training.
Innovative Methods
Regulations9 call for simulator manufacturers to develop practical and cost-effective stall recognition and recovery training that will transfer positively to real airplanes. One problem, however, is the lack of aircraft manufacturersâ data needed to modify simulator aerodynamic models. Developing and validating these models for all simulator types is impractical. Therefore, flight simulator manufacturers must revert to innovative stall modeling methods that provide a viable solution to the problem â without requiring the use of actual aircraft stall flight data. One such method allows for the use of existing engineering data to create a stall model for the type of aircraft being simulated, providing representative stall and post-stall flight characteristics.10 The models can be implemented in an existing training device and after successful evaluation by test pilots.
An example is a âblack boxâ approach called StallBox, which was developed and tested by Bihrle Applied Research.11 As shown in the diagram, StallBox connects to the original equipment manufacturer simulator model and blends type-representative stall characteristics as an extension to that model.
After StallBox was developed, testing and evaluation were performed by test pilots and airline pilots to compare the stall recognition and recovery characteristics of the type-representative stall model, a stall model using type experimental data, and the unmodified baseline simulator model.12 The pilots had wide-ranging opinions on some stall characteristics such as buffet, roll off and high-altitude stall recovery technique, but after testing was completed, they said in a presentation to an American Institute of Aeronautics and Astronautics conference that they agreed that âin situations when developing a stall model based on flight data is not practical, the alternative approach of developing a model based on computational aerodynamics, wind tunnel data and subject expert opinion appears feasible.â
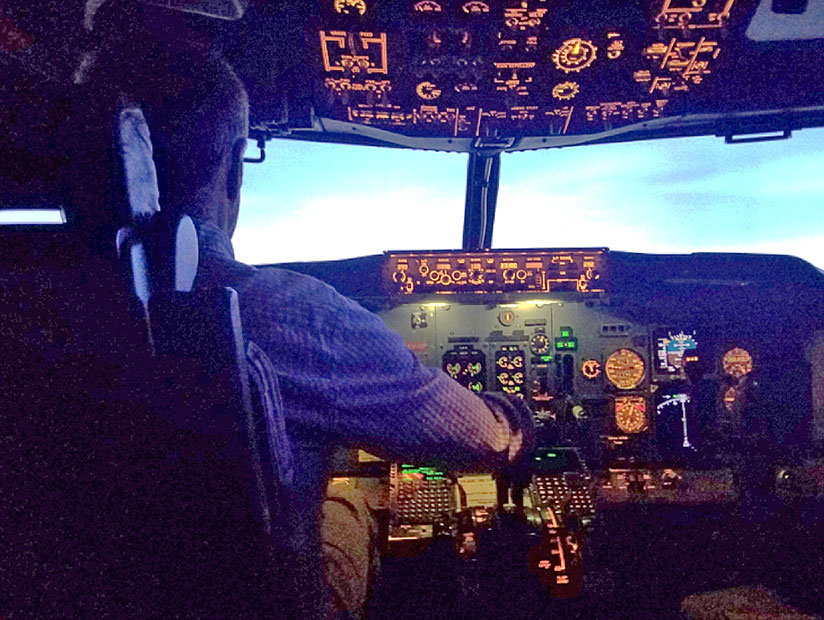
FSTD Limitations
An upset is defined by the International Civil Aviation Organization (ICAO) âas an airplane in flight unintentionally exceeding the parameters normally experienced in line operations or training, normally defined by the existence of at least one of the following parameters:13
- âPitch attitude greater than 25 degrees, nose upâ;
- âPitch attitude greater than 10 degrees, nose downâ;
- âBank angle greater than 45 degreesâ; or,
- âWithin the above parameters, but flying at airspeeds inappropriate for the conditions. â
For all normal flight conditions, FSTDs provide an adequate platform for effecting positive habit transfer to the airplane. However, that is not entirely the case for upset conditions, which are characterized by large amplitude pitching, rolling and yawing motions. Additionally, FSTD limitations in presenting realistic inertial and motion cues can affect the pilotâs perception of control response and the result of control inputs. To minimize the risk of negative habit transfer, the FAA emphasizes the importance of not using an FSTD in upset prevention and recovery training (UPRT) if the simulator responds differently than the airplane.14 Therefore, effective UPRT performed in an FSTD must consider its limitations and the effects of those limitations on human perception and performance.
UPRT via In-Flight Simulators
In-flight simulation (IFS) aircraft such as the highly modified Calspan Aerospace Learjet have been extensively employed for UPRT.15 The aircraft has dual control systems â a baseline Learjet flight control system and a computer-driven system that can host a variety of aircraft models, just as with an FSTD. When the simulation system is engaged, the aircraft is âflownâ by an evaluation pilot, who experiences the handling characteristics of the airplane being simulated. A safety pilot monitors the flight and can disengage the system if there is a safety of flight issue or malfunction. Advantages of using an IFS aircraft for conducting UPRT include the absence of scaling of motion cues and the ability to readily simulate upset-causing control malfunctions. Disadvantages include cost and limited aircraft availability.
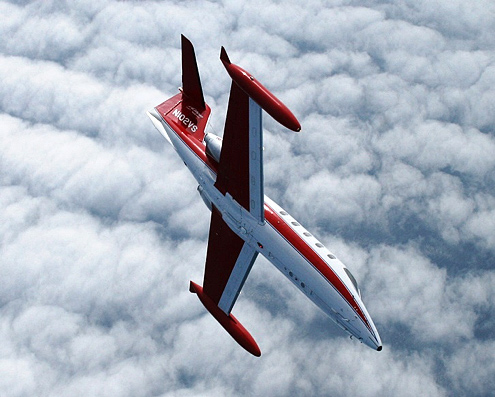
An evaluation of stall and upset recovery training was sponsored by the U.S. Naval Air Systems Command in Patuxent River, Maryland, and performed by Calspan Aerospace using its IFS Learjet, and NASA using its cockpit motion simulator fitted with an integrated flight deck (IFD). The purpose was to evaluate an integrated training program including the IFS and ground based simulators for UPRT training.16 The same upset maneuvers were performed by a group of test pilots and line pilots in the IFS Learjet and in NASAâs IFD. The IFD motion algorithm was modified for the evaluation with enhanced stall buffet cueing.
In all, 13 UPRT tasks were performed by the pilot participants, beginning with some basic IFS and IFD familiarization. The tasks involved upsets in all three axes, including nose-high and nose-low recoveries, upsets due to trim and control system malfunctions, and control problems. Half the pilots began their evaluations in the IFD, and the other half, in the IFS. Pilots who began in the IFD felt it prepared them better for the flight in the IFS, while pilots who trained first in the IFS were ambivalent but nevertheless felt that the realism of training in the aircraft first established appropriate expectations for training in the ground-based IFD. Assessing these results, it is important to remember that both the IFD and the IFS used essentially the same simulation model. The only differences were the motion and visual cues. Of note, most pilots felt that training in the IFS for UPRT was the most valuable.
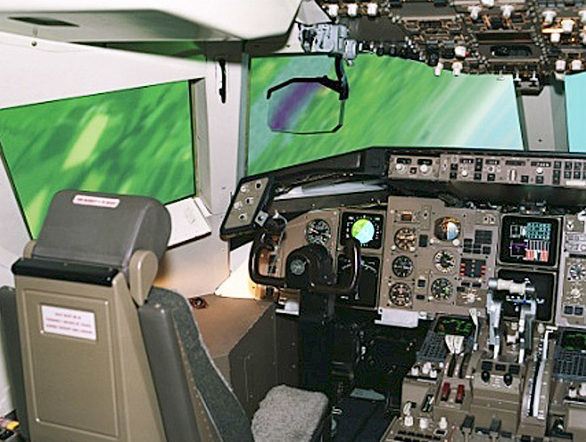
The new pilot training requirements to mitigate LOC-I using FSTDs alone will not provide the necessary motion and gravitational acceleration (g) cues that a pilot must correctly perceive to diagnose and recover from real-world upsets. If the training is not representative of real-world conditions, it can result in negative habit transfer.
However, the Calspan-NASA research on the use of ground-based simulators for UPRT17 found that initial training in an FSTD helped pilots perform better when first exposed to real flight upset conditions in the IFS. In fact, pilots receiving this training did consistently better on their final evaluation flights in the IFS.
Effective training can be accomplished in a ground-based simulator. But as explained in the research, âwhile FFS (FSTD) training did result in a significant improvement in pilot skill, knowledge and confidence, pilots were not able to reach the satisfactory level of proficiency from FFS (FSTD) training alone. The IFS-trained group, on the other hand, was able to fully assimilate the maneuver cues and use that information to develop and implement a successful recovery strategy.â
Until simulator technology finds a way of representing perceptual cues that are critical to a pilotâs state awareness and control performance, their use to satisfy the new training rules will likely be limited.
Richard J. Ranaudo was a NASA research pilot for 25 years and the lead project test pilot in the icing research program for 16 years. After retiring from NASA, he spent five years as manager of Canadair flight test programs and conducted icing development and certification testing on prototype business and regional aircraft.
Notes
- CFI Notebook.net. âRetention and Transfer of Learning.â
- FAA. âPilot Certification and Qualification Requirements for Air Carrier Operations.â
- Six degrees of freedom refers to the freedom of movement of a rigid object in three-dimensional space. The six degrees are forward and backward, up and down, and left and right.
- Wikipedia. âSimulator Sickness.â
- Stephens, C.; Harrivel, A.; Prinzel, L.; Comstock, Ray A.; Abraham, Nijo; Pope, Alan; Wilkerson, James; Kiggins, Daniel C., âState Monitoring and Line-Oriented Flight Training for Attention Management,â International Symposium on Aviation Psychology, (ISAP 2017), 19th, May 8â11, 2017, Dayton, Ohio, U.S.
- NTSB. Aircraft Accident Report NTSB/AAR-96/01, âIn-Flight Icing Encounter and Loss of Control; Simmons Airlines, d.b.a. American Eagle, Flight 4184; Avions de Transport Regional (ATR) Model 72-212, N401AM; Roselawn, Indiana; October 31, 1994.â July 9, 1996. The crash killed all 68 passengers and crew and destroyed the airplane. The NTSB said the probable cause of the accident was âthe loss of control, attributed to a sudden and unexpected aileron hinge moment reversal, that occurred after a ridge of ice accreted beyond the deice boots while the airplane was in a holding pattern during which it intermittently encountered supercooled cloud and drizzle/rain drops, the size and water content of which exceeded those described in the icing certification envelope. The airplane was susceptible to this loss of control, and the crew was unable to recover.â
- NTSB. Aircraft Accident Report NTSB/AAR-10/01, âLoss of Control on Approach; Colgan Air Inc.; Operating as Continental Connection Flight 3407; Bombardier DHC-8-400, N200WQ; Clarence Center, New York; February 12, 2009.” Feb. 2, 2010. All 49 passengers and crew and one person on the ground were killed in the crash, which destroyed the airplane. The NTSB said the probable cause was âthe captain’s inappropriate response to the activation of the stick shaker, which led to an aerodynamic stall from which the airplane did not recover.â
- FAA Flight Standards Service, National Simulator Program. NSP GB 14-01, FSTD Qualification Guidance Bulletin. April 11, 2016. Washington.
- FARs Part 60 Change 2, Subchapter D, âFlight Simulation Training Device Initial and Continuing Qualification and Use.â
- Personal conversation with D.G. Gingras, Bihrle Applied Research.
- Gingras. D.R.; Ralston, J. N.; Oltman, R.; Wilkening, C.; Watts, R.;Â Desrochers, P. AIAA 2014-1003, âFlight Simulator Augmentation for Stall and Upset Training.â AIAA Modeling and Simulation Technologies Conference, Jan. 13â17, 2014, National Harbor, Maryland, U.S.
- Schroeder, J.; BĂŒrki-Cohen, J.; Shikany, D.A.; Gingras, D. R.; Desrochers, P. AIAA 2014-1002, âAn Evaluation of Several Stall Models for Commercial Transport Training.â AIAA Modeling and Simulation Technologies Conference, Jan. 13â17, 2014, National Harbor, Maryland, U.S.
- ICAO. Document 1011, âManual on Aeroplane Upset and Recovery Training,â Section I, First Edition. 2014.
- FAA. Advisory Circular 121-111, âUpset Prevention and Recovery Training,â Change 1, Paragraph 2-5a. Jan. 4, 2017.
- Calspan Advanced Maneuvering and Upset Recovery Training Course, Buffalo, New York, U.S.
- Donaldson, S.; Priest, J.; Cunningham, K.; Foster, J.V. NF1676L-15115, âUpset Simulation and Training Initiatives for U.S. Navy Commercial Derived Aircraft.â AIAA Modeling and Simulation Technologies Conference, Aug. 13â16, 2012.
- Ibid.
Image credits
Featured image: © SuperJet International | Wikimedia CC-BY-SA 2.0
NASA test aircraft: U.S. National Aeronautics and Space Administration
Flight simulation training device: © Xinhua | Alamy Stock Photo
Stallbox diagram and photo: © Bihrle Applied Research
Learjet: © Calspan
NASA IFD: U.S. National Aeronautics and Space Administration